Research projects
Understanding the replication machinery of bunyaviruses
The order of Bunyavirales is a highly diverse group of viruses including numerous human pathogens. The WHO R&D Blueprint emphasizes the urgent need for medical countermeasures against several bunyaviruses, including the Lassa virus, Crimean-Congo hemorrhagic fever virus and Rift Valley fever virus. These viruses are a threat to global health and the economy especially in low- and middle-income countries but they are also recognized for their high epidemic potential and, due to climate change, their expanding geographic distribution. Many of these viruses must be handled in biocontainments of the highest biosafety standards limiting the method and technology spectrum for research. Despite the severe diseases they can cause, bunyaviruses are fascinatingly simple: they contain a segmented negative strand RNA genome and produce only a handful of own proteins, some of them get by with only four gene products.
Two proteins are mainly in the focus of our research: The large (L) protein and the nucleoprotein N that together with the RNA genome of the virus form the ribonucleoparticle, which is sufficient for replication and transcription of the genome. We apply a variety of methods from structural biology, biochemistry and virology fields to understand bunyavirus genome replication and transcription processes in tiny detail. These processes lead to production of new viral proteins in cells (viral transcription) as well as the amplification of the viral genome (genome replication) to finally assemble new viral particles, ready to infect the next cells. The key player in these processes is the viral L protein, which is a multifunctional and highly optimized molecular machine, comparable to a swiss army knife. Our goal is to understand this molecular machine - its functions, its regulation, its interactors - to finally develop strategies to inhibit the processes of genome replication and transcription and thereby stop bunyavirus amplification.
In the following, we will introduce our research questions and describe selected publications in more detail.
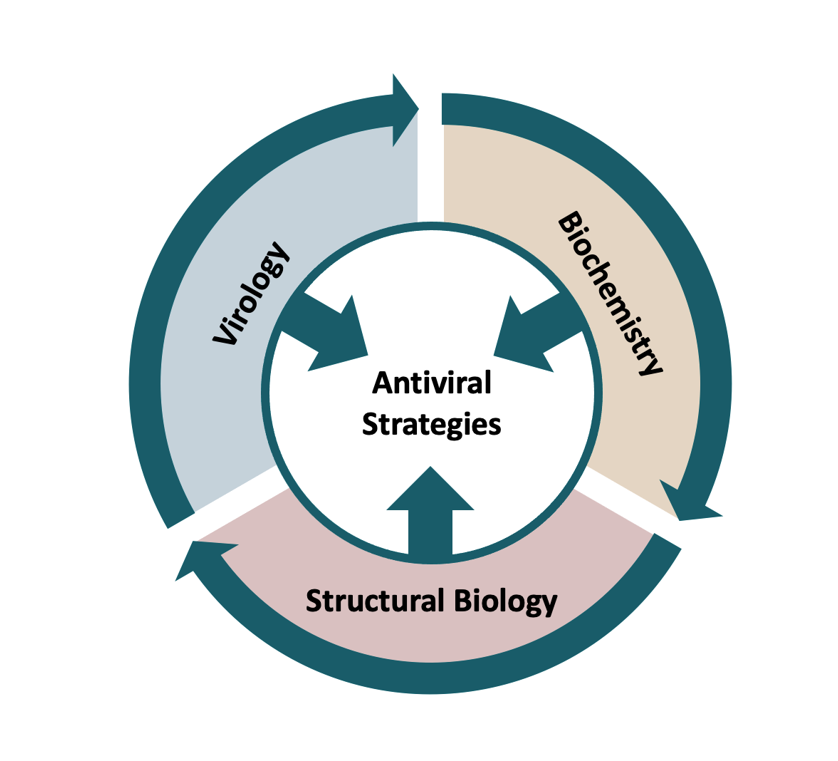
The cap-snatching mechanism of bunyaviruses
Viruses have developed different strategies to produce their viral proteins within the host cell. Bunyaviruses use a cap-snatching mechanism to initiate viral transcription - meaning they steal the cap from the host cell to produce a viral transcript which is then translated into viral proteins by the host cell machinery. During cap-snatching, a short nucleotide sequence is cleaved from the 5’ end of the host mRNA and used to prime viral RNA synthesis. While this process is well understood for influenza virus, our knowledge on how exactly this works in bunyaviruses is still very limited.
In 2020, we published a review article providing an overview of the recent structural and functional data of the cap-snatching mechanism of bunyaviruses. We described similarities and differences between the nuclear-replicating influenza virus and the cytoplasmically replicating bunyaviruses, and highlighted open questions for the bunyaviral cap-snatching mechanism: (1) Which cellular capped mRNAs are targeted? (2) How do bunyaviruses bind the cap? (3) Where exactly in the cytoplasm do bunyaviruses perform cap-snatching? (4) And how do bunyaviruses assure the viral mRNAs reach the ribosomes to be translated into viral proteins?
Structure of a functional cap-binding domain in Rift Valley fever virus L protein
In the process of cap-snatching, a short capped RNA fragment is cleaved off host cell mRNA and subsequently used to prime viral mRNA synthesis. Thus, both an endonuclease activity to cleavage RNA as well as a cap-binding activity to sequester the host cell mRNA cap are required. Accordingly, influenza virus cap-snatching involves an endonuclease domain and a cap-binding domain which are both located within the polymerase complex. While an endonuclease had indeed been identified in the bunyavirus L protein, no functional cap-binding domain had been reported. In the past years, we obtained evidence for a functional cap-binding domain within bunyavirus L protein by solving crystal structures of isolated domains of the L protein as well as biophysical assays verifying a specific interaction between the protein and the ligand. Our crystal structure demonstrated a very similar architecture of the cap-binding domains of certain bunyaviruses and influenza viruses with a central β-sheet packed against a long α-helix. The cap-ligand is sandwiched between two aromatic side chains.
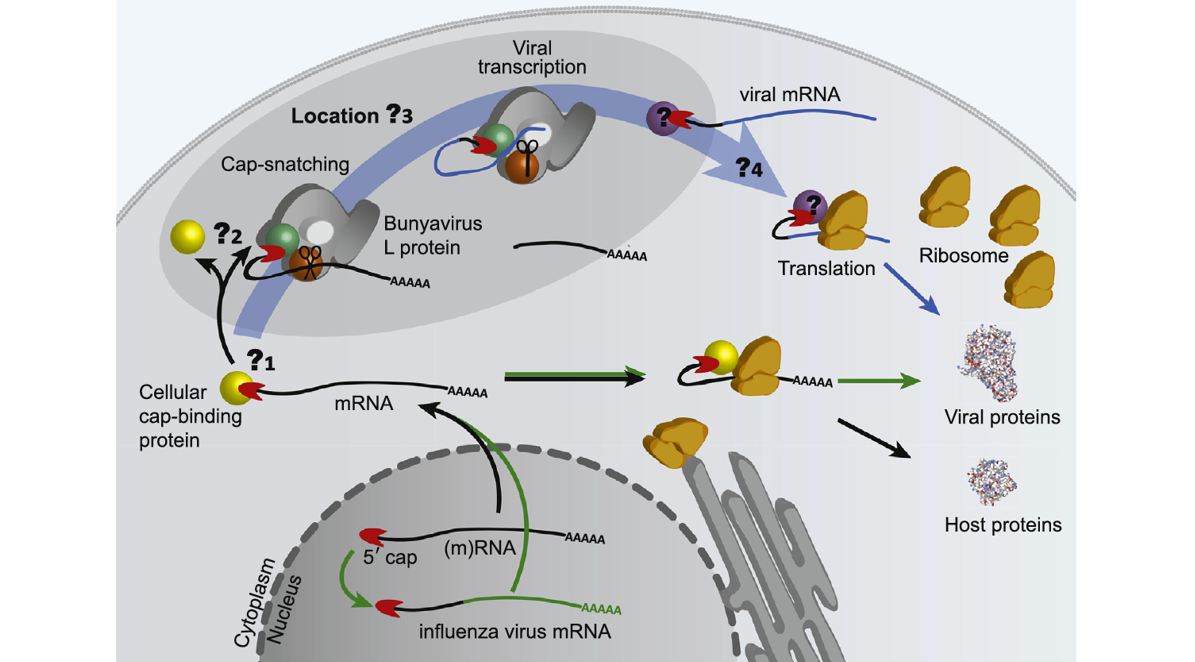
Publication:
Olschewski-Pavlita S, Cusack S, Rosenthal M. The Cap-Snatching Mechanism of Bunyaviruses. Trends Microbiol. 2020;28(4):293-303. doi:10.1016/j.tim.2019.12.006
An RNA to rule them all: Critical steps in Lassa virus ribonucleoparticle assembly and recruitment
Viral proteins have to interact with each other, with host cell proteins as well as viral RNA and other biomolecules in a targeted manner to ensure successful amplification of the virus. We are using native mass spectrometry as a method to analyze protein-protein and protein-RNA interactions in bunyaviruses. This method allows to “weigh” proteins in their natural state. We aim to understand how viral ribonucleoparticles (RNP) are assembled and recruited to the viral assembly site. The RNP is an important part of the virus as it is the minimal unit for viral genome replication and transcription in cells (see above). We discovered that when the nucleoprotein N encounters small pieces of RNA, it changes its shape and interactions with other N proteins causing the originally trimeric ring-like N complex to dissociate into monomers attached to the RNA. These RNA-bound N proteins then form larger assemblies reminiscent of RNPs. Additionally, we found that another viral protein, called Z protein, directly interacts with N in both the presence and absence of RNA. This interaction is important for RNP recruitment to viral assembly sites within cells. Interestingly, the strength of this N-Z interaction is reduced in a more acidic environment, similar to the conditions when the virus enters our cells, which may facilitate the release of the RNPs from the viral envelope upon infection of new cells. Understanding these mechanisms of RNP formation and recruitment is important for the identification of potential targets for antiviral intervention.
Publication: Saenger L., Williams HM, Yu D., Vogel D. Kosinski J, Rosenthal M*, Uetrecht C*. An RNA to rule them all: Critical steps in Lassa virus ribonucleoparticle assembly and recruitment. BioRxiv DOI: 10.1101/2023.02.09.527830
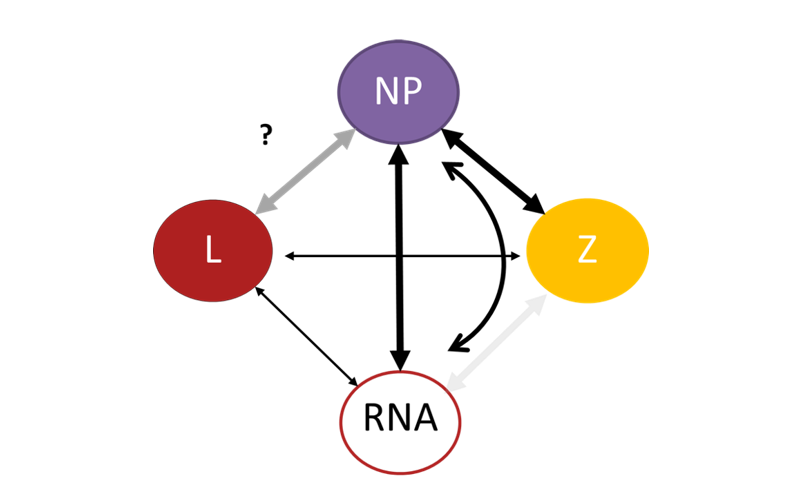
Biochemical characterization of the Lassa virus L protein
For in vitro studies we produce the bunyavirus L protein in insect cells using baculoviruses. This allows us to investigate how this multidomain and multifunctional protein replicates the viral genome and produces viral mRNA in vitro on a fundamental molecular level. We established different biochemical assays allowing us to study the multiple functions of L.
LASV L protein synthesizes RNA - both initiated by a primer and in an unprimed (de novo) manner. For RNA synthesis L requires manganese rather than magnesium ions, as commonly seen in other polymerases. Experiments with various short primers revealed the presence of two RNA replication initiation sites on the template as well as primer translocation as proposed by the prime-and-realign hypothesis for de novo initiation of RNA synthesis. Furthermore, we showed that RNA synthesis can be inhibited by the LASV Z protein.
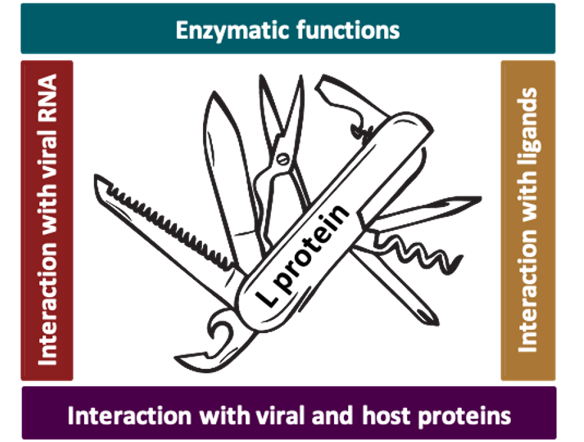
Publication:
Vogel D, Rosenthal M, Gogrefe N, Reindl S, Günther S. Biochemical characterization of the Lassa virus L protein. J Biol Chem. 2019 Mar 29. pii: jbc.RA118.006973. doi: 10.1074/jbc.RA118.006973
Kouba T, Vogel D, Thorkelsson SR, Quemin ERJ, Williams HM, Milewski M, Busch C, Günther S, Grünewald K, Rosenthal M*, Cusack S*. Conformational changes in Lassa virus L protein associated with promoter binding and RNA synthesis activity. Nat Commun. 2021 Dec 2;12(1):7018. doi: 10.1038/s41467-021-27305-5
Conformational changes in Lassa virus L protein associated with promoter binding and RNA synthesis activity
Using single-particle cryo-EM, we determined nine 3D structures of the LASV L protein. Each structure shows the LASV L protein in a different conformational state, illustrating its need for flexibility in order to fulfill its multiple functions. This high flexibility is the reason why the structure determination was so challenging. Since flexible regions move relatively fast, they are very difficult to image and appear blurred or are not visible at all. Our extensive biochemical characterization of the LASV L protein allowed us to stabilize the L protein in several functionally relevant conformations of genome replication. This way we were able to get high-resolution structural information on how the LASV L protein is regulated by protein-RNA interactions. Overall, we determined structures of the L protein in the apo-, promoter-bound pre-initiation and active RNA synthesis states. We characterized distinct binding pockets for the conserved 3’- and 5’-promoter RNAs and showed how full promoter binding induces a distinct pre-initiation conformation in which the 3’-RNA terminus points into the active site of the RNA-dependent RNA polymerase (RdRP). In the apo- and early elongation states, the endonuclease is inhibited by two distinct peptides from within the L protein, whereas in the pre-initiation state, it is uninhibited. In the early elongation state, a template-product duplex is bound in the active site cavity together with an incoming non-hydrolysable nucleotide, and the entire C-terminal region of the L protein, including the putative cap-binding domain, is well-ordered. These data advance our mechanistic understanding of how this flexible and multifunctional molecular machine is activated.
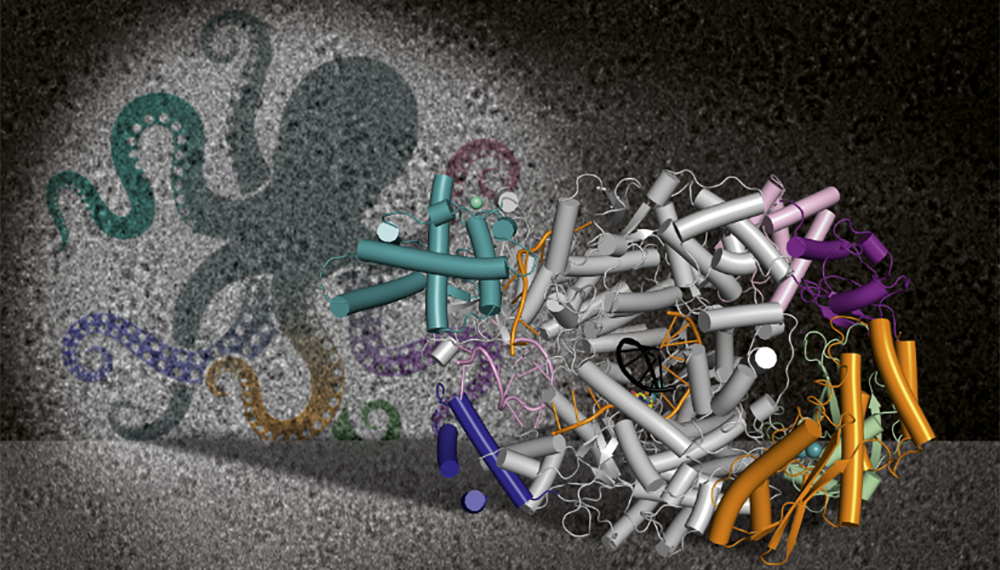
Structural and functional insights into the severe fever with thrombocytopenia syndrome virus L protein
In 2020, we determined the first 3D structure of the severe fever with thrombocytopenia syndrome virus (SFTSV) L protein using single-particle electron cryo-microscopy (cryo-EM). This initial structure allowed us to determine the overall conformation of the SFTSV L protein, which comprises several functional domains, including an N-terminal endonuclease, an RNA-dependent RNA-polymerase (RdRp), and a C-terminal capped-RNA binding domain. We next determined a series of high-resolution structures of the SFTSV L protein, also via cryo-EM, representing several states of the genome replication process, from pre-initiation to late-stage elongation. From this data, we could identify how the SFTSV L protein binds the 5′ viral RNA in a hook-like conformation (see illustration on the right) and show how the distal 5′ and 3′ RNA ends form a duplex positioning the 3′ RNA terminus in the active site of the RdRp ready for initiation. We also observed the L protein stalled in the early and late stages of elongation, which allowed us to identify the channels by which newly-synthesized RNA and spent template RNA exit the SFTSV L protein core.
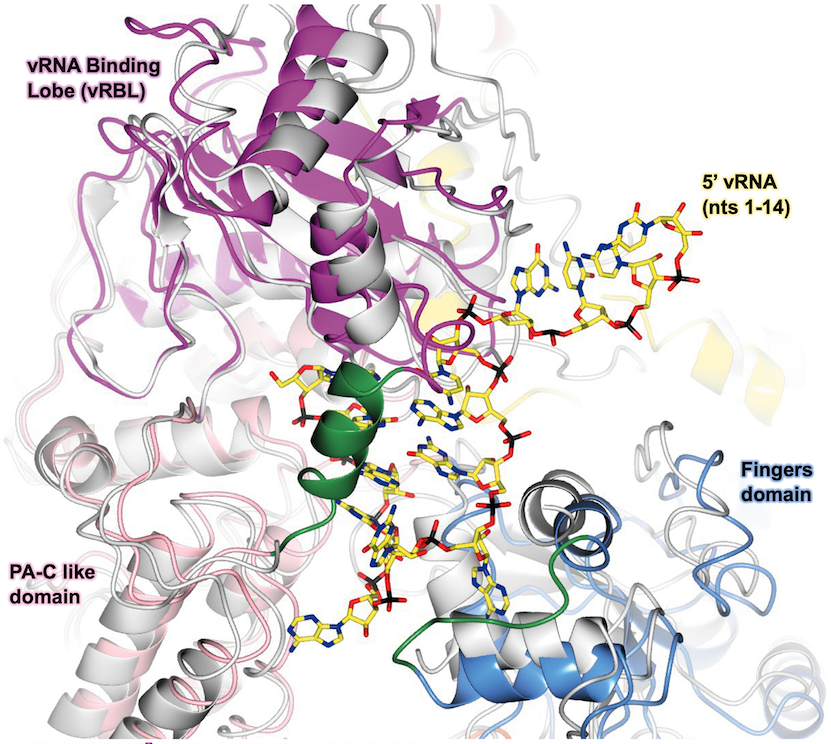
Publications:
Vogel, D., Thorkelsson, S.R., Quemin, E.R.J., Meier, K., Kouba, T., Gogrefe, N., Busch, C., Reindl, S., Günther, S., Cusack, S., Grünewald, K., and Rosenthal, M., 2020. Structural and functional characterisation of the severe fever with thrombocytopenia syndrome virus L protein. Nucleic Acids Research, doi: 10.1093/nar/gkaa253
Williams, H.M., Thorkelsson, S.R., Vogel, D., Milewski, M., Busch, C., Cusack, S., Grünewald, K., Quemin, E.R.J., and Rosenthal, M., 2023. Structural insights into genome replication by the severe fever with thrombocytopenia syndrome virus L protein. Nucleic Acids Research, doi: 10.1093/nar/gkac1249
Structural and functional characterization of the Sin Nombre virus L protein
Hantaviruses are zoonotic viruses that are globally distributed and can cause severe disease in humans. One important example is Sin Nombre virus (SNV), which is the most prevalent human-pathogenic hantavirus in North America. To this day, there are no effective vaccines or specific FDA-approved therapeutics for the treatment of hantavirus infections. The targeted search for inhibitors of viral genome replication and transcription requires a profound knowledge of both the structure and function of the multifunctional L protein. We established protocols for the expression and purification of the SNV L protein as well as different biochemical assays to assess its key functions: (i) RNA degradation catalyzed by the endonuclease, (ii) binding to the viral RNA promoter, and (iii) RNA synthesis catalyzed by the RdRp. Additionally, we used single-particle cryo-EM to obtain a structural model of the L protein core region, including the RdRp, in complex with the viral 5′ promoter RNA.
Publications:
Meier K, Thorkelsson SR, Durieux Trouilleton Q, Vogel D, Yu D, Kosinski J, Cusack S, Malet H, Grünewald K, Quemin ERJ, Rosenthal M. Structural and functional characterization of the Sin Nombre virus L protein. PLoS Pathog. 2023 Aug 7;19(8):e1011533. doi: 10.1371/journal.ppat.1011533
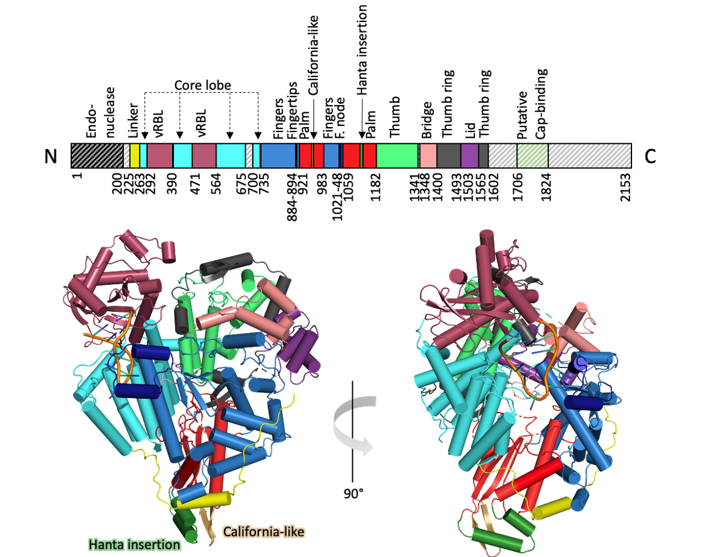
Translating insights from basic research into antiviral strategies
We used cryo-EM to solve several L protein structures in complex with viral RNA. We characterized the RNA-binding and enzymatic functions of L using biochemical assays and cell-based studies to identify regulatory elements. We now evaluate the suitability of the different L protein functions and regulatory mechanisms such as the RdRp, secondary RNA binding sites, and the cap-binding site as potential targets for antiviral approaches. To this end, in collaboration with the Fraunhofer Institute for Translational Medicine and Pharmacology (ITMP, ScreeningPort Hamburg) we develop robust, high-throughput-feasible assays to measure these functions and screen small molecule libraries. Hit compounds are validated in counter assays and cell-based assays and further analyzed regarding the structure-activity relationship. In vitro assay formats we use include fluorescence polarization, homogeneous time-resolved FRET, surface plasmon resonance, microscale thermophoresis and viral minireplicon assays. This project translates knowledge from the academic basic research conducted at BNITM into antiviral strategies in collaboration with our partners from the Fraunhofer ITMP.
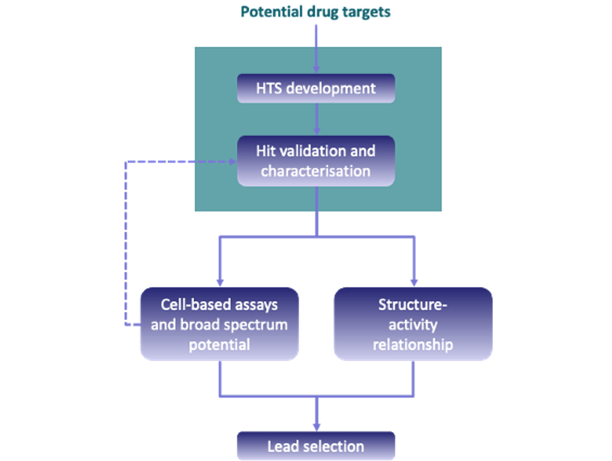